Should Your System Use Synthetic or “Hot Fluid” Thermal Fluid?
By: Michael R. Damiani Radco Industries, Inc.
Published in the October 1998 issue of Process Heating magazine
High temperature heat transfer fluids are used in process applications where their optimum bulk fluid operating temperatures of 300°F to 750°F are safer and more efficient than steam, electrical, or direct fire heating methods. Selecting the proper heat transfer fluid for a new system while in the design phase or for possible process fluid improvements during an upcoming retrofit will assure sufficient and uniform BTU delivery (or removal). The properly selected heat transfer fluid will also minimize potential production loss and downtime due to required design changes, mechanical problems, or fluid failure. The process of selecting the optimum heat transfer fluid should begin once the energy transfer required by the process and the planned/actual service ratings of the mechanical components of the heat transfer system have been calculated and thoroughly researched. Since there are a number companies specializing in heat transfer fluids and a wide range of fluid products available, the knowledge of this key element of the system’s operating requirements can help to create a set of criteria that can be used to compare various fluids and allow rapid elimination of fluids that are not best suited for the application. However, before comparing and contrasting various individual fluids, much time and effort in the selection process can be saved by comparing and contrasting the various chemistries of the fluids. Once a fluid chemistry is selected that best meets the performance properties and other criteria required by the application, the resultant list of potential fluids becomes significantly more manageable for more detailed apples-to-apples comparisons.
Fluid Chemistry. High temperature heat transfer fluids can be categorized by chemical structure into three primary groups:
1. Synthetics
2. Hot Oils
3. “Others” including silicones
The synthetics, also referred to as “aromatics”, consist of benzene-based structures and include the diphenyl oxide/biphenyl fluids, the diphenylethanes, dibenzyltoluenes, and terphenyls. Depending on the specific product, the overall bulk fluid temperature operating range of the synthetics is from -70°F to 750°F.
The hot oils are petroleum-based and most consist of paraffinic and/or napthenic hydrocarbons. The overall bulk fluid temperature operating range of the petroleum-based fluids is from -10°F to 600°F, with the high-grade hydrogenated white oils strongly recommended for applications requiring bulk fluid temperatures in the 575°F to 600°F range.
Silicone-based fluids, and to a greater extent hybrid glycol fluids, are used primarily in specialized applications requiring process/product compatibility should a heat exchanger leak occur. This group’s performance and cost factor disadvantages in the comparative temperature ranges of the synthetics and hot oils make silicone-based and other specialty fluids unlikely choices for most process applications.
Fluids and System Types. Hot oils and synthetics are used in a multitude of heat processing applications. The type of system design used in the process is a major consideration on the choice of a specific fluid chemistry. Processes utilizing heat transfer fluids can be categorized into three system types:
1. Non-pressurized liquid phase systems.
2. Pressurized liquid phase systems.
3. Pressurized pumped or natural circulation vapor-phase systems.
Non-pressurized liquid phase systems are generally the simplest to design and operate. Both hot oils and synthetics can be used equally well in this type of system as long as the operating temperature of the heat transfer fluid is below its boiling range. Major components in these systems consist of the heater, heat exchanger, vented expansion tank, and circulating pump. The expansion tank need not have inert gas applied in these types of systems in order to keep positive pressure on the circulating pump. To reduce the probability of fluid oxidation, a baffled expansion tank design is preferred to assure the fluid is below 350°F at the fluid/atmosphere interface.
Pressurized liquid phase systems using both hot oils and synthetics are similar in design to non-pressurized systems except that inert gas is applied through the expansion tank when the required operating temperature of the heat transfer fluid is above its boiling range. The pressurized inert gas (nitrogen) is used to maintain the heat transfer fluid as a liquid. The inert gas also acts as a buffer in the expansion tank between the hot fluid surface and the atmosphere, eliminating fluid oxidation. With the exception of the multi-phase fluids like the diphenyl oxide/biphenyl-type, most of the liquid phase synthetics and all of the hot oils do not require inert gas pressurization to maintain the liquid phase at their top-end recommended operating temperatures.
Pressurized vapor-phase systems utilize only a handful of synthetic fluids, most notably the diphenyl oxide/biphenyl-type. A simple vapor phase system can be designed using hydrostatic pressure to gravity return the condensate from the user to the vaporizer, eliminating the need for a condensate pump. More complex systems require a flash tank, condensate return tank, and a condensate return pump. The disadvantage of the added capital equipment cost and the complexity of vapor phase systems is offset by the increased BTUs delivered per pound of vapor versus liquid, and increased temperature control at the user- important in heat sensitive processes.
Criteria For Selecting The Best Fluid Chemistry. If a existing system or a system on the design board is a vapor phase system and requires a high temperature fluid, the fluid options are extremely limited. Only a handful of high temperature vapor phase synthetic fluids are available from different heat transfer fluid manufacturers. Hot oils cannot be used in the vapor phase. Therefore, non-pressurized or pressurized liquid phase systems allow the greatest variance in potential end-use fluids, both synthetic and petroleum-based. Especially in existing systems, the wide range of new liquid phase fluid technology available offers possibilities of increased system performance and energy savings with a minimum of downtime and cost. Whether researching a potential fluid upgrade in an existing system or specifying the proper fluid type and fluid into a new design, the following basic criteria should be considered:
Thermal Stability. Thermal stability is simply defined as the inherent ability of a heat transfer fluid to withstand molecular cracking from heat stress. Relative thermal stability testing of heat transfer fluids measures a particular fluid’s molecular bond strength at a specific temperature versus another particular heat transfer fluid at the same temperature and under identical testing conditions. ‘Relative’ is the key word- since the tests are run under ideal laboratory conditions and do not factor in real-world fluid stresses such as mechanical malfunctions, design flaws, oxidation, etc., the data generated is useful for comparative purposes only. Accurate predictions of fluid life in actual processing applications should not be implied from thermal stability data.
A fluid’s thermal stability is the primary factor in determining its maximum bulk fluid operating temperature. This is the maximum temperature the fluid manufacturer recommends the fluid can be used and still maintain an acceptable level of thermal stability. Since fluid degradation rates are closely tied to temperature, continuous use above the manufacturer’s recommend maximum bulk fluid operating temperature will increase degradation rates exponentially. Potential system problems caused by excessive degradation and the subsequent formation of degradation by-products include increased coking and fouling, mechanical difficulties, and decreased heat transfer efficiency. Therefore, in selecting a fluid chemistry, the first step in the selection process is to determine the maximum bulk operating temperature required by the process. Most hot oils have a recommended maximum bulk fluid temperature of 550°F – 600°F, while the aromatics have recommended maximum bulk fluid temperatures between 600°F- 750°F, depending on the fluid. Since the molecular structures of the aromatics are significantly more thermally stable than the hot oils above 600°F, in applications above this temperature aromatic-based heat transfer fluids are strongly recommended. Process applications requiring bulk fluid temperatures from 300°F to 600°F can specify either synthetic or petroleum-based fluids. Within this temperature range relative thermal stability data supplied from fluid manufacturers is available to compare individual fluids at specific temperatures.
Heat Transfer Efficiency. Heat transfer efficiency comparisons between heat transfer fluids are made using heat transfer coefficients. At a specific temperature, a fluid’s overall heat transfer coefficient can be calculated using its density, viscosity, thermal conductivity and specific heat at a determined flow velocity and pipe diameter. The resultant heat transfer coefficients may be then evaluated and compared. At a given temperature, the heat transfer coefficients of the fluid types may differ as much as 30%. Depending on the thermal resistance factors of the other components in the system, a fluid with a substantial heat transfer coefficient advantage may allow a reduction in sizing of system equipment. Replacing existing heat transfer fluid with a more efficient heat fluid may significantly increase production output and/or reduce energy costs. Most of the aromatic-based fluids have a significant advantage in heat transfer efficiency over hot oils from 300°F to 500°F. Above this temperature range (up to 600°F) petroleum fluids narrow the difference somewhat with a select number of highly refined paraffinic/napthenic white oils having a slight efficiency advantage over the mid-range aromatics.
Keep in mind the heat transfer coefficient is calculated using virgin fluid properties. Fluid that has been in service for an extended period of time and has undergone thermal degradation may have a significantly lower coefficient due to fluid viscosity changes and the presence of less efficient fluid degradation by-products. Therefore, a fluid’s thermal stability plays an important role in maintaining its thermal efficiency over time.
Pumpability Point. Pumpability point, not freeze point, is the true low-end temperature a heat transfer fluid can operate in heat process applications. The pumpability point is defined as the temperature at which the viscosity of the fluid reaches a point (typically 2000 cP) where centrifugal pumps can no longer circulate the fluid. Although most high temperature process applications run at bulk temperatures well above hot oil and aromatic pumpability points, system designs that might encounter cold weather during emergency shutdowns, maintenance shutdowns, or operate a batch process in a cold climate, should take into account fluid pumpability points. Generally, most of the hot oils offer adequate start-up protection down to the 0°F to +25°F range. The mid-temperature aromatics (with 650°F maximum bulk temperatures) offer protection down to -70°F to -20°F, while the top-end temperature aromatics (with 700°F- 750°F maximum bulk temperatures) are at +40°F to +60°F. Processes using a fluid that potentially may have start-up problems in cold weather will need to be heat traced.
Fluid Serviceability. Fluid replacement, reprocessing, or filtration may be required from time to time due to unexpected temperature excursions, system upsets, or contamination. Because of the relatively low cost of petroleum-based fluids, very few suppliers offer reprocessing services for hot oils or a credit program for the off-spec material that can be applied toward the cost of a new charge. Most synthetics are composed of a limited number of aromatic components and have a narrow boiling range, allowing easy identification of degradation by-products and/or contaminants. Reprocessing synthetics using fractional distillation is an economical alternative to disposal and replacement; hence, most synthetic fluid suppliers offer this service at a nominal cost. Some synthetic fluid suppliers also offer credit for off-spec material- this credit is then applied to the purchase cost of the replacement charge. These programs eliminate fluid turnaround and reprocessing time, since the new fluid can be charged in immediately after the off-spec fluid has been drained. This is especially useful if the system downtime is unscheduled or a short maintenance period has been planned.
Filtration versus reprocessing or fluid replacement is a cost effective method of removing carbon and coke suspended in the heat transfer fluid. Most fluid suppliers recommend slipstream filter loops permanently installed and closely maintained on both hot oil and synthetic systems. Fluid filtering companies with portable units are also available to setup on-site and remove carbon from the fluid while the unit is still operating. Almost all suppliers of both synthetic and petroleum-based heat transfer fluids offer analytical testing of fluid condition at no-charge. This important service monitors fluid condition over time and gives an early warning should action need to be taken to replace, reprocess, or filter the fluid.
Environmental. Comparing environmental and personnel guidelines is just as important when selecting a heat transfer fluid chemistry as comparing fluid performance. In general, all heat transfer fluids do not present an appreciable health hazard when used in accordance with acceptable manufacturing practices. However, the petroleum-based fluids offer substantial advantages in ease of handling, reprocessing, shipping and disposal as compared to the synthetics. For one, most hot oils do not have a reportable spill quantity, and in the cases of the white mineral oils, meet the FDA and USDA criteria for ‘incidental food contact’. Also, the petroleum-based fluids do not form hazardous degradation by-products, therefore most spent hot oils can be sent to a local oil/lube recycler for disposal. Finally, the hot oils tend to warrant no special handling precautions, are not DOT regulated, and require no special storage requirements. From a personnel standpoint, the hot oils are extremely user-friendly. Most have a non-discernible odor and are non-toxic both in contact with skin and ingestion.
Because of the aromatic-based chemistry of most of the synthetics, some fluids can form hazardous degradation by-products that require special permits, handling and shipping precautions. Some synthetics and their vapors may cause skin and eye irritation after prolonged exposure, and emit pungent odors. In some cases, spills of synthetic fluids require reporting under the Superfund Amendments and Re-authorization Act. Since there is a wide range of chemistries available within the aromatic group, not all fluids have similar properties and environmental/personnel concerns. Regulations and precautions vary from fluid to fluid.
Cost. As a general rule, the higher the bulk fluid temperature a fluid is rated, the higher the cost of the fluid. The synthetics rated for use above 650°F are two to three times more expensive than the average hot oil rated to 600°F, while aromatics rated from 600°F to 650°F are one and a half to two times the cost of the average hot oil.
Which Chemistry Is The Best? Chances are one fluid chemistry is not superior to the other in every criteria required by a new process or retrofit. Both fluid chemistries have advantages- the aromatics offer superior heat transfer efficiency and stability at elevated temperatures coupled with serviceability and adequate pumpability, while the hot oils have a significant cost and environmental/personnel advantage. Identification of the primary criteria required by a new process or the main improvement goal desired in a retrofit will prioritize the criteria by importance. Is the goal more output and/or shorter production runs? A personnel/environmentally friendly system? Longer period of time between shutdown and fluid replacement? By first selecting the fluid chemistry that best solves the big picture, comparisons of individual fluids within the group should solve the little ones.
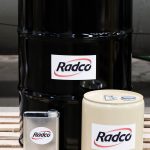